Blog Material Applications: High-Temperature Corrosion
By: Dave Olsen
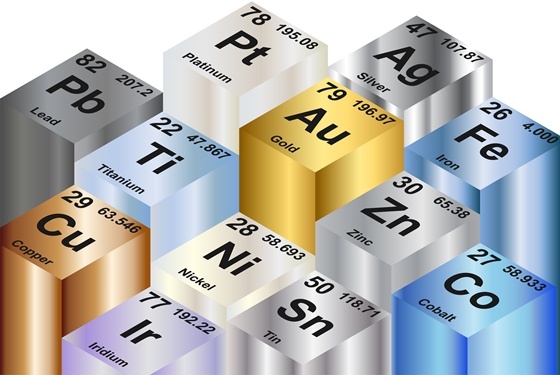
The Effect of Temperature on Corrosion of Metals
As the performance demands on metals tend to increase as temperature increases, so do the types of corrosive attacks to which the metal is likely to be subjected. When we think of significant industries and applications that are most likely to face the combined effects of high temperature with a corrosive environment significant ones come to mind:
- Gas and Steam Turbines
- Heat Treating
- Mineral Processing
- Chemical Processing
- Pulp and Paper
- Waste Incineration
- Fossil Fuel Power Generation
High-temperature corrosion performance is a form of corrosion that does not require the presence of a liquid electrolyte. Some important forms of high-temperature corrosion to consider that often cause equipment problems are:
- Ash/Salt Deposit Corrosion
- Carburization
- Halogen Corrosion
- Metal Dusting
- Molten Metal Corrosion
- Molten Salt Corrosion
- Nitridation
- Oxidation
- Sulfidation
Environments are often classified as either oxidizing or reducing, depending on the level of oxygen present. An oxidizing atmosphere generally contains excess free oxygen; a reducing atmosphere generally has very low oxygen activity. Reducing atmospheres generally increase the corrosive effect of sulfidation, carburization, nitridation, or ash/salt deposit corrosion. Hot corrosion and fuel/ash corrosion are usually related to liquid salt deposits that destroy the protective oxide scale on the metal surface.
Ash/Salt Deposit Corrosion
In some industrial processes, ashes and salts are deposited on surfaces of process components. In certain cases, the deposit is a scale which leads to reactions between the ash/salt and the protective oxide layer on the metal alloy, or otherwise reduces the oxide layer. When the oxide layer reforms, a little more of the substrate metal is consumed. Any breakdown of that protective oxide layer reduces the material’s resistance to corrosion, and/or consumes metal and reduces wall thickness, eventually leading to failure.
Carburization
Carburizing results from exposure of metal to carbon-containing environments including CO, CH4, or other hydrocarbon gases at elevated temperatures. Carburization often causes the alloy to experience embrittlement or degradation of other mechanical properties. Process and petrochemical piping are examples of where design and material accommodation must be made for the potential development of carburization.
Inclusion of chromium or nickel in the alloy helps to create a barrier that inhibits carbon-infusion into the metal. Silicon creates an SiO2 layer that can limit carbon infusion, and niobium can be used to create a carbide that ties up carbon atoms in a stable form.
Sometimes materials are intentionally carburized to increase hardness and wear resistance. That process does come at a cost over time as certain mechanical properties are reduced and material is consumed.
Halogen Corrosion
Many metals react readily with halogen gases (fluorine, chlorine, bromine, iodine, and astatine) at elevated temperatures to form volatile metal halides. These materials can suffer severe high-temperature corrosion. Industrial processes where fuels or feedstocks are contaminated with impurities such as sodium or potassium allow halogens to react with these impurities to form NaCl and KCl. Stainless steels are very susceptible to corrosion in fluorine gas at temperatures as low as 570°F/300°C.
Metal Dusting
Metal dusting is a form of carburization that occurs in high temperature environments where carbon is present in forms including CO, CH4, or other hydrocarbon gases. It is common in equipment used in heat-treating, such as furnace retorts, baskets, fans, etc. Severe attacks may occur after a year in service, often at the interface between metal and refractories. The result is pitting of the metal and thinning of sections.
Alloys that have been found to suffer metal dusting include carbon steels, Cr-Mo steels, stainless steels, Fe-Ni-Cr alloys, and iron- and nickel-based alloys. Copper, silicon and chromium, and aluminum are commonly added to alloys to provide metal dusting resistance. There are tradeoffs, as the addition of alloying elements adds to cost and can result in the loss of some mechanical properties.
Molten Metal Corrosion
Liquid metals are often used as heat transfer media (the use of liquid sodium in nuclear reactors, for example). The containment material, which is in contact with the molten metal, is subject to molten metal corrosion. It is best to consider how molten metals will react with the material in the containment system. For example, molten aluminum attacks iron-, nickel- and cobalt-base alloys. Molten zinc or lead reacts with nickel-base alloys, but molten lead may be contained by cast iron, steels, and stainless steels.
Molten Salt Corrosion
Molten salts are used in industries such as heat treating, nuclear energy, concentrated solar power (where molten salt provides thermal storage and heat transfer), and the extraction of reactive metals. Like Molten Metal Corrosion, the containment material, which is in contact with the molten salt, is subject to corrosion. Molten salts remove the oxide scale from metal surfaces, so corrosion may take the form of uniform thinning, pitting, or inter-granular attack.
Nitridation
Metals are susceptible to nitridation when exposed to ammonia-bearing or nitrogen-based environments at elevated temperatures. During nitridation, the alloy absorbs nitrogen from the environment and results in a metal that is hard and brittle, with a loss of metal and strength.
Austenitic stainless steels have been used for processing equipment in ammonia-bearing environments. If stainless steels are attacked due to severe environments (higher temperatures or higher ammonia concentrations, for example) nickel-based alloys may be used. Nickel is the most effective alloying element in improving nitridation resistance. Ceramics may be a selected alternative to metal in these applications, but lack ductility and are difficult to fabricate. When controlled, though, the resulting hardness, and wear resistance caused by nitridation, may be beneficial.
Oxidation
Oxidation is the most important high-temperature corrosion reaction and is often present even when other forms of corrosion dominate. The creation of an oxide layer generates a protective scale that helps resist many types of corrosion. So reducing environments – where there is no excess oxygen available – hinder a metal’s tendency to generate the oxide scale and are generally more susceptible to corrosion. The rate of oxidation for metals increases as temperature increases.
Many oxidation problems are caused when an alloy is used in a temperature range exceeding its capability. Oxidation of this type may form scaling and internal voids that affect the integrity and structural capability of a material.
Oxidation in air may occur in many industrial processes. For example, heat treat furnaces are often heated by electrical resistance, so that alloys are oxidized by the oxygen in the air. In many industrial processes, heat is generated by combustion, such as mixing air with natural gas. Alloys in these environments may be oxidized by oxygen in the air, but other products of combustion such as H2O and CO2 may also play a part in affecting the oxidation reaction.
Sulfidation
Sulfur is generally present as a natural occurring impurity in crude oil. When combustion occurs with excess air, sulfur in the fuel reacts with oxygen to form SO2 or SO3. Sulfidation corrosion results in the thinning of walls in components, tubing or piping, or of pressure vessels. Failure of these components can be catastrophic with the rupture releasing large volumes of combustible gas.
In most cases, metals rely on an oxide scale to resist sulfidation. Combustion environments generated by incinerating waste may contain sulfur, chlorine, zinc, lead and potassium. At incineration temperatures alloys that are resistant to both sulfidation and chloride attack are needed.
Conclusion
Differing environments contribute to an alloy’s corrosion resistance at elevated temperatures. Often a material that performs well against certain types of attack will underperform against others. So a solid understanding of the greater environment will help make the best high performance cost-effective material choice.